Plate tectonics is the theory proposing that Earth’s outer rocky shell is divided into seven major and several smaller rigid plates. Forces generated by heat losses from the planet’s interior constantly move the plates about. Plate movements, ongoing over millions of years (see Geological History), open and close ocean basins, generate volcanoes, raise mountains, facilitate accumulation of mineral and petroleum deposits, and influence evolution and climate change. Friction between plates prevents steady motion and stores energy that is released in sudden movements, causing earthquakes.
This article is the full-length entry about plate tectonics. For a plain-language summary, please see Plate Tectonics (Plain-Language Summary).
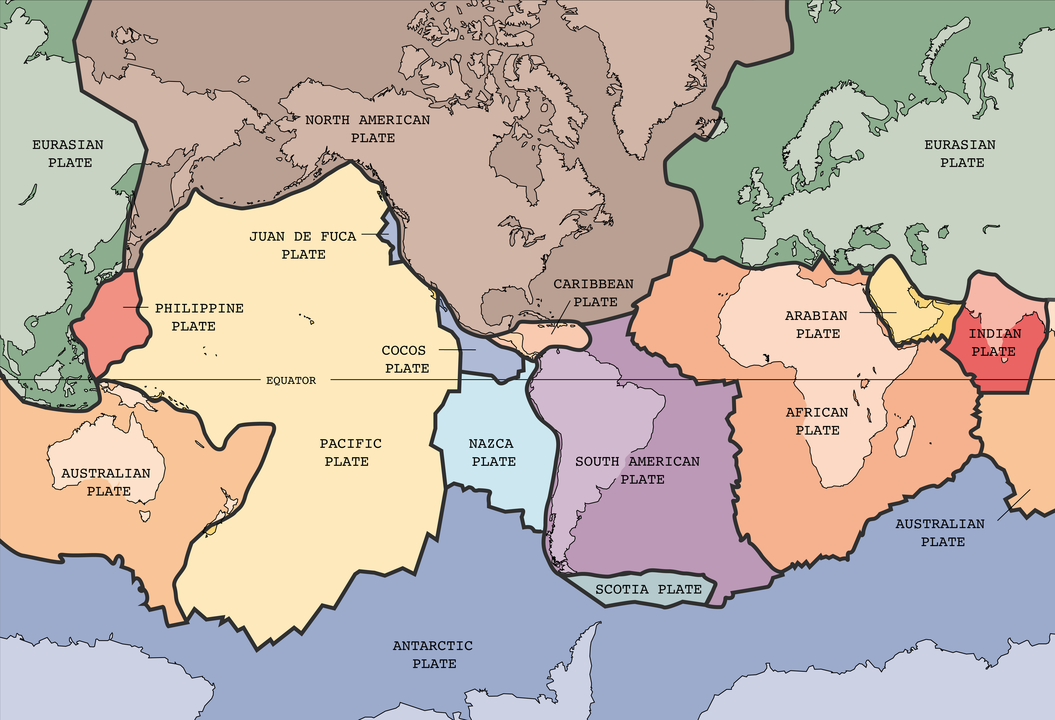
History
Early Discoveries and Theories
When early European explorers visited the Americas some 500 years ago, they observed that the coasts of South America and Africa would fit together closely if they were not separated by the Atlantic Ocean. At the time, it was believed that Earth was about 6,000 years old. Therefore, it was thought that any separation must have occurred as the result of cataclysmic events at the time of creation.
During the 19th century, geologists suggested that to provide enough time for the great thicknesses of sedimentary rock to have accumulated, Earth must be older than 6,000 years, perhaps hundreds of millions of years old. Conversely, physicists argued that Earth was losing heat to space and that, since no adequate sources of internal energy were known, it must be cooling. They pointed out that, even if Earth had been red-hot when formed, it would have cooled to its present temperature in a few tens of millions of years. They also held that as Earth cooled, contraction wrinkled its surface like the skin of a drying apple, the wrinkles being mountain belts. In 1896, the discovery of radioactivity led to the realization that radioactive elements are widespread in rocks and could provide an internal heat source. The discovery also led to ways of determining Earth’s age at about 4.6 billion years.
Continental Drift
In 1910, American geologist Frank B. Taylor suggested that movements of the continents gave rise to Earth’s great mountain belts. In 1912, Alfred Wegener, a German meteorologist, proposed that all continents were merged into a single supercontinent called Pangea, which broke apart about 200 million years ago. Since then the continents have been moving separately through the ocean floors like ships. This theory became known as continental drift. At a major conference in 1926, most authorities rejected Wegener’s ideas largely because there was no mechanism known that would allow continents to move through ocean floors. A few supporters held out in areas where evidence for drift was strongest, such as the Alps and South Africa. In the latter, Alexander L. du Toit proposed that Pangea had broken into Gondwanaland in the south and Laurasia in the north before fragmenting into existing continents.
Seismology and Earth’s Interior
Nothing has done more to increase our understanding of Earth as a planet than the study of earthquakes, called seismology (see Geology). Earthquakes were a familiar but little understood phenomenon until, in the late 19th century, the emperor of Japan invited John Milne from England to study them. Milne and his contemporaries produced the first modern seismographs, the first worldwide network for reporting earthquakes and a theory to explain earthquake waves. The improved seismographs enabled studies by French father and son Edmond and Jean-Pierre Rothé, as well as the Americans Beno Gutenberg and Charles F. Richter. These scientists showed that most earthquakes follow the principal mountains and island areas around the Pacific Ocean and the Himalayan and Alpine ranges across Eurasia.
The velocities of seismic waves depend on rock and mineral compositions and on changes caused by increasing temperature and pressure with depth. Knowing this, seismology also revealed the nature of Earth’s internal structure. The Earth’s internal structure can be visualized as a soft-boiled egg: the shell is a cool, brittle crust of visible rocks, beneath this is a white, thicker mantle of denser rocks, and beneath this the “yolk,” i.e. a core rich in liquid iron.
The existence of a readily deformable layer at a depth of a few tens of kilometres beneath the surface is well illustrated in north-central North America in different ways. For example, it is illustrated by the depression beneath the load of glaciers during the recent ice ages. It is also illustrated by the uplift of the land since the ice sheets melted (see Glaciation). The uplift has left beaches raised by hundreds of metres around the shores of the Great Lakes and Hudson Bay.
Scientists recognized that material could move laterally out from beneath the load of ice, much as water is displaced by a boat, and return when the load was removed. This realization led to a second set of terms for Earth’s outer rock layers based on rock strength. The relatively cool, stiff and mechanically strong outermost rocky layer is called the lithosphere (rock sphere). It includes the crust and uppermost parts of the Earth’s mantle. In thickness the lithosphere varies from over 200 km beneath the old, cold parts of continents, such as the Canadian Shield, to a few kilometres below parts of the ocean floors. Gradationally below this is the asthenosphere (weak sphere). It is the mechanically weak, ductile layer mostly in the upper mantle that can flow at rates of a few centimetres per year.
Ocean Floors
The collection of information about the deep ocean floors began in the mid-19th century, when Commodore Matthew F. Maury, US Navy, made soundings across the Atlantic Ocean. These soundings revealed that the central part is shallower than the rest. The Challenger Expedition (1872-76) showed this shallower region was part of a great ridge, called the Mid-Atlantic Ridge. The ridge lay along the axis of the ocean. Later expeditions found ridges in other oceans. These expeditions located deep trenches off the continental coasts and the convex sides of arcuate volcanic island chains around the Pacific Ocean.
Enough was known, even in 1910, for American geologist Frank Taylor to propose that the Mid-Atlantic Ridge was a rift with the crust moving away from it on both sides. In the 1920s, Canadian geologist Reginald A. Daly speculated that the mountain chains of the western Americas were caused by the sliding of the continental masses towards the Pacific, with the Atlantic opening behind. In England in 1931, Arthur Holmes suggested that radioactive heating caused convection currents within Earth’s mantle. These currents rose beneath the ocean floor, spread out in opposite directions and eventually descended at the trenches. Unfortunately, until instruments were developed to permit detailed measurements, little firm evidence was available to permit general acceptance of these ideas.
This changed, starting with echo sounders in the 1930s and towed magnetometers used to detect submarines during the Second World War. These innovations allowed ocean floors to be mapped in detail by remote sensing. In 1956, Maurice Ewing and Bruce Heezen of Columbia University noticed that some earthquakes coincided with the crests of known oceanic ridges. By 1960, they had established the existence of Earth’s longest mountain chain, winding its way for 60,000 km down the axis of the Atlantic Ocean, around South Africa to the middle of the Indian Ocean. One branch follows the Gulf of Aden northward into the Red Sea and another passes south of Australia and New Zealand to cross the Pacific and join the San Andreas Fault at the head of the Gulf of California. The ridge rises to form islands such as Iceland, the Azores and Easter Island.
Great Faults and Fracture Zones
Californian oceanographers observed that the crests of oceanic ridges were intersected at intervals. The ridges were also offset by as much as hundreds of kilometres on great fracture zones that resembled faults. In addition, the ridges appeared to end abruptly at continents. These submarine features added fuel to arguments about observed amounts of offset on huge faults recognized on land, such as that recognized in 1936 by Canadian Clifford H. Stockwell near Great Slave Lake.
In 1946, geologists in Scotland and New Zealand proposed that huge faults with lateral offsets of over 100 km crossed those countries, beginning and ending in the sea. In 1953, California geologists showed that rocks on either side of the San Andreas Fault, which began in the Gulf of California and ended in the Pacific off Cape Mendocino, were offset from one another by 560 km. These discoveries posed a problem for believers in a rigid Earth, because they could not explain such large offsets or how such large faults could be terminated.
Rock Magnetism
Earth’s magnetic poles coincide approximately with the geographic poles of rotation that locate the axis upon which Earth spins. Early in the 20th century it was demonstrated that many rocks acquired a weak magnetization at the time they formed, a force they retain permanently. It is believed that the Earth’s magnetic field imparts this magnetization.
The inclination of the magnetic field varies systematically from steep at high latitudes to shallow at low latitudes. Because of this, measurement of the magnetic direction within a rock potentially can locate the position of the magnetic pole at the time the rock formed.
In 1954, British geophysicist Keith Runcorn found that pole positions obtained from rocks of different age plotted at different locations along what became known as a polar-wandering curve. Further work showed that polar-wandering curves from other continents were different from one another. However, if the positions of the continents were restored to something like those suggested by Wegener, the curves came together. Their discovery provided quantitative evidence for continental drift and inaugurated a gradual change of opinion. Over the next two decades, most Earth scientists came to accept continental drift, although a mechanism for moving continents still had to be found.
Early studies showed that some rocks were magnetized in a reverse direction from the present magnetic field. In the 1920s, Japanese geophysicist Motonori Matuyama recognized that reversals were of different ages. Periodically, the north magnetic pole becomes the south magnetic pole and vice versa. Reversal ages are now well known and occur at irregular intervals from a few thousand to millions of years, with about 200 happening in the last 100 million years. In 1961, American Arthur Raff and Englishman Ronald Mason published a map of the ocean floor off the west coast of North America between latitudes 52º and 40º. The map featured orderly patterns of stripes of higher magnetic intensity alternating with ones of low intensity. It also showed the symmetry of stripes on opposite sides of a ridge that was segmented and offset by transverse fracture zones. This map was seminal in formulation of plate tectonic theory.
Overview of Plate Tectonics
The essential difference between the theories of plate tectonics and continental drift is that the latter assumed continents moved through the ocean floors. According to plate tectonics, both continents and ocean floors are integral parts of the “cracked egg-shell” of rigid but mobile lithospheric plates that overlie the ductile asthenosphere. Knowledge about the nature of the ocean floors — which occupy over 60 per cent of Earth’s surface — was the major contributor to the theory. Plates have three kinds of boundaries: divergent plate, transform fault and convergent plate.
Divergent Plate Boundaries
Spreading ridges, or divergent plate boundaries, are places where plates move apart and grow by addition of basalt from the mantle. In 1960, American Harry H. Hess elaborated on Holmes’ idea of mantle convection cells. He suggested that the ascending arm of a convection cell rises beneath the ridge in the eastern Pacific Ocean and splits the ridge apart. This allows basaltic lava to extrude and solidify to form new ocean floor. The ocean floors on either side of the ridge move away to eventually sink at the deep ocean trenches and disappear into the mantle beneath the mountain belts and island arcs bordering the Pacific.
In 1963, Canadians Lawrence W. Morley and Andre Larochelle first showed that long lines of magnetic anomalies form parallel stripes situated symmetrically along both sides of the crest of the Mid-Atlantic Ridge. They realized that the stripes were caused by reversals in Earth’s magnetic field. Their ideas seemed so strange that two reputable journals rejected their paper. However, later that year, Fred Vine and Drummond H. Matthews in Britain published similar observations from the Indian Ocean. They also interpreted these observations as the result of reversals in Earth’s field. As basaltic lava extrudes along the rift at the ridge crest and solidifies, it “freezes in” the direction of the ambient magnetic field. Therefore spreading is recorded by the “bar code” of normal and reversed magnetic anomalies on the ocean floor. This result was quickly accepted with ocean floor mapping supplemented in 1968 by the Deep Sea Drilling Program in the United States. The program collected rock samples from the ocean floor for analysis and isotopic dating. The oldest ocean floor is a little less than 200 million years old and located near the Mariana Islands in the western Pacific Ocean.
Transform Fault Boundaries
The second type of plate boundary occurs where plates slide past one another along transform faults. A plot of earthquake locations superimposed on the sea-floor map off the North American Pacific coast, noted earlier, demonstrated this. It showed that the southern tip of the spreading ridge that lay offshore between northernmost California and southwest British Columbia is connected via the San Andreas Fault to the main Pacific spreading ridge off Central America. Hence, the spreading of the ridge system evidently controls lateral movement on the connecting fault.
The connection of one spreading ridge to another via an active fault led John Tuzo Wilson in 1965 to recognize that this was a special kind of fault. He called it a transform fault because it transforms one kind of plate boundary into another. It explains the origin of the great fracture zones that cross ocean floors. For example, in the Atlantic the mid-ocean ridge does not follow the same curved shape as the coasts of Brazil and Africa. Instead, it is broken into a series of short segments, each fairly straight and each end of which is offset by a fracture zone that ends abruptly at the continents. The spreading ridges form at right angles to the direction of separation of the continents. They are broken and offset from one another at intervals by fracture zones that form parallel to the motion of the continents. Only those parts of the fracture zone that connect the spreading ridges are active and generate earthquakes. Their inactive continuations, between ridge and continent, record earlier spreading back to initial separation of the continents. The segmentation of the oceanic crust along spreading ridges and transform faults reflect accommodation of the blocks of growing oceanic floor to the spherical surface of Earth as the Americas rotated away from Africa and Eurasia.
Convergent Plate Boundaries
Third, convergent plate boundaries are where plates come together and one plate dives beneath another along a subduction zone to disappear into the mantle. Before the Second World War, Kiyoo Wadati in Japan and Hugo Benioff in California located the positions of earthquake sources, or foci. Their locations were beneath the chains of volcanic islands in the western Pacific Ocean and the mountains bordering the eastern Pacific. The foci define surfaces that extend downward from the oceanic trenches to depths of as much as 700 km, and dip away from the ocean at angles ranging from about 65º in the western Pacific to 30º below the mountains. The earthquakes apparently were generated by movement on gigantic reverse faults as the Pacific Ocean floor was overridden by surrounding regions.
Ocean floor mapping shows that over the last 90 million years an average of 3.4 km2 per year has been added to Earth’s surface area at spreading ridges. Because Earth evidently is not swelling like an inflating balloon, the enormous volume of new lithosphere generated must be balanced by the return of a comparable amount to the mantle. As ocean floors move away from spreading ridges at rates of between mostly three and 10 cm per year they cool by conduction at the rock-water interface. As they cool, they gradually become denser and subside so that ocean floors can be thought of as the top surfaces of giant convection cells. Eventually the oceanic lithosphere becomes so dense that it sinks at the oceanic trenches into the mantle along subduction zones. These are the descending limbs of the convection cells, located by the reverse faults recognized by Wadati and Benioff.
Because of convective circulation, ocean floors are constantly being renewed. As continental rocks are less dense and more buoyant than those of ocean floors, the continents are not readily subducted. Instead, they are moved around Earth’s surface on constantly growing and disappearing oceanic conveyer belts, like scum in a pot of boiling dirty water. This provides explanations for “continental drift.” It also explains why continents contain many rocks up to 2.5 billion years old and even a few nearly 4 billion years old, whereas no ocean floors are older than 200 million years.
As the ocean floor starts to subduct, in places material is scraped off and accreted to the overriding plate to form distinctive bodies of disrupted ocean floor rocks, which underlie much of New Zealand, parts of Japan, California and southern Alaska. Elsewhere, the base of the overriding plate may be thinned by a process called subduction erosion. As ocean floor rocks continue to sink along subduction zones to depths of about 100 to 250 km, they release superheated water. This water rises and lowers the melting point of rocks in the overlying lithosphere. Molten rocks that reach the surface form the chains of volcanoes around the Pacific Ocean that give rise to the name Ring of Fire. Those rocks that cool and solidify in the crust form great bodies of granitic rock such as those in the Coast Mountains of British Columbia. The rising heat also thins and weakens the lithosphere, so that under lateral compression it can be deformed and thickened to build mountain chains like the Andes and Cordillera. The depth to which subducting rocks sink is debated. Some suggest that it is only to the 700 km depth recorded by earthquakes. Others use a technique called seismic tomography. This technique is based on the fact that the rates of seismic wave transmission are faster in the cooler subducting slab than the enclosing hot mantle. Seismic tomographic images show plates descending to near the core-mantle boundary. Ocean floor rocks that 100 million years ago were disappearing beneath western North America now lie 2,800 km below the eastern seaboard of the continent.
The former Tethys Ocean, that once lay between the Indian and Eurasian continents, closed over 40 million years ago. It did so by subduction beneath what is now Tibet. When this occurred, collision between the buoyant continents piled up the Himalayas and raised the Tibetan Plateau. How then did the North American Cordillera and the Andes form when there were no continental collisions, merely the Americas overriding Pacific Ocean lithosphere? In places, fragments of mainly former volcanic island chains are embedded in the mountains, but none are large enough to have raised continental-scale mountain belts. Mountain building was initiated when the Atlantic Ocean started to open, and it seems that the westward drive of the Americas was so rapid that Pacific lithosphere could not subduct fast enough to get out of the way. Because of this the continent in effect collided with the ocean floor, much as suggested by some early “continental drifters.” This is happening today, where the Pacific Plate dives northward beneath Alaska and raises the highest mountains in North America.
Rifting
In 1930, German geologist Hans Cloos drew attention to the association of faults, earthquakes and volcanoes along the Rhine and East African rift valleys. Volcanism and upward doming are greatest at triple points, places where three plates meet. For example, the Ethiopian highlands are where the Red Sea, Gulf of Aden and East African Rift valley come together. They are linked by the Red Sea, which has opened in the last 10 million years to the mountains of Sinai where the north end of the Red Sea and gulfs of Suez and Aqaba meet. This relationship suggests that oceans start to form where rifts connect a series of volcanic centres and that the rifts spread to form widening oceans. When this happens a fourth type of boundary is created within a plate along the boundary between high-standing continent and low-standing ocean floor. Here great thicknesses of sediment eroded from the continent are deposited on the adjacent ocean floor. Today, such deposits lie off the eastern seaboard of North America, where rifting that started some 200 million years ago led to opening of the North Atlantic Ocean. An ancient example of such a boundary, formed between about 500 and 200 million years ago, is preserved in the Canadian Rocky Mountains.
Hot Spots
Hot spots form above cylindrical columns. The columns have mushroom-shaped heads called mantle plumes that rise beneath oceans and continents by convection originating from deep within the mantle. Some, like Hawaii or Yellowstone, are isolated within plates, Others, like Iceland and Tristan da Cunha, lie along mid-ocean ridges. At surface, hot spots are marked by extrusion of voluminous amounts of lava, and associated with trails of extinct volcanic islands or lava fields. These become progressively older away from the active hot spot and form as the lithosphere moves across the head of the mantle plume. A single trail extends north north-westward for over 30º of latitude from Hawaii and formed as the Pacific Plate moved in that direction over the plume. A double trail forms the Walvis and Rio Grande ridges in the South Atlantic Ocean. These ridges extend from the Tristan da Cunha islands above the present plume head to the coasts of Africa and South America. Here, volcanic rocks 130 million years old mark the ends of the trails. This relationship supports the view that 130 million years ago a hot spot formed beneath Gondwana and, linked by rift valleys to other hot spots, eventually determined the location of the Atlantic Ocean.
Present System of Plates
Earth’s surface is broken into seven major plates and many smaller ones. The Pacific Plate is the largest. It consists entirely of oceanic lithosphere with the exception of Baja California in Mexico and southwestern California. By comparison, the African, Antarctic, Eurasian, Indian and North and South American plates contain both continental and oceanic lithosphere.
The pattern is slowly, but ceaselessly, changing. Most changes are gradual, but occasional plate reorganizations take place. One may be happening today within the Indian Ocean in reaction to the 40 million year long collision between India and Tibet. Another may be happening in the geologically-rapid changing geography of southeastern Asia. Here, the continent of Australia, part of the Indian Plate, drives northward into the Indonesian complex of volcanic islands, continental fragments and small ocean basins. No parts of the Atlantic or Indian oceans are more than 180 million years old. The Red Sea and Gulf of Aden are about 10 million years old. The East African Rift valleys are younger still and are only beginning to open. Even though the Pacific Ocean is spreading at a rate three times that of the Atlantic, it is diminishing in area as it is being overridden by surrounding plates.
Earlier Cycles of Ocean and Mountain Building
The present oceans, with the exception of the Pacific, mark only the last part of a cycle that began when the supercontinent Pangea broke apart 200 million years ago. The ancestor of the Pacific was the “superocean” called Panthalassa. Panthalassa occupied over half Earth’s surface when Pangea existed. If the two sides of the North Atlantic are reassembled, it is clear that the Scandinavian, Scottish, Appalachian and Moroccan mountains once formed a single chain in the heart of Pangea. This chain was created when former oceans closed, only to open later, roughly in the same place, to form the present Atlantic.
Between 1.3 billion and 900 million years ago, all continents were assembled into a supercontinent called Rodinia. Rodinia began to break up about 750 million years ago into continents that then recombined about 300 million years ago into Pangea. The cyclic opening and closing of ocean basins has been called the Wilson Cycle by Kevin Burke and William Kidd. The linear patterns and associations of rocks characteristic of those formed during plate tectonic processes are different in the Archean eon, before about 2.5 billion years ago, indicating that tectonic processes operating then were different.
Consequences of Plate Tectonic Activity
Aspects of plate tectonics of direct concern are earthquakes, tsunamis and volcanoes. Most of these events are generated at plate boundaries. Earthquakes happen all the time, but the largest are generated where plates come together. Friction causes converging plates to stick; stress accumulates over periods that may amount to hundreds of years until the stored energy is suddenly released in a major earthquake. There is evidence for a succession of big earthquakes just offshore between northern California and northern Vancouver Island. Here the small Juan de Fuca Plate is being overridden by the big North American Plate. The last major earthquake in this area was in 1700. Such earthquakes may cause the seabed and coast to suddenly subside or rise and the displaced seawater to generate tsunamis. The type of volcanic activity that often accompanies plate convergence may be explosive and destructive, as in the 1980 eruption of Mount St. Helens, Oregon.
On the positive side, many kinds of mineral deposits are generated by plate tectonic activity. Hot springs carrying metal sulphides accompany spreading ridges. When the hot water enters cold seawater, sulphides are precipitated as lead and zinc and sulphide ores around the spring. The chains of volcanic and plutonic rocks above subduction zones host widespread deposits of copper, molybdenum and gold, such as those mined in the Cordillera and Andes. During mountain building, any fluids containing metal sulphides may be squeezed laterally and concentrated in chemically favourable settings. The thick sedimentary deposits formed within plates at continent-ocean boundaries, such as those flanking the Atlantic Ocean, contain accumulations of hydrocarbons.
Collision between continents allows life forms to migrate from one continent to another and, with subsequent continental separation, to evolve independently into different species. The movement of continents causes climates to change and is at least partly responsible for periodic ice ages. In some continental configurations, ocean currents that flow between warm equatorial regions and cool high-latitude regions distribute heat and give rise to equable climates. In other configurations, such as Antarctica, which is almost entirely surrounded by spreading ridges, currents flow around it so the continent loses heat and an ice age results.